Table of Contents
Chemistry is once again taking a front seat in the quest to find ways to manufacture new, green, people-friendly, sustainable, multifunctional complex molecules for an array of practical implementations.
One method highlighted in this resurgence uses drop-in building blocks to provide a faster way to integrate biomass feedstocks without the need to drastically change high-capital-investment production processes. However, many of these monomers require ultraviolet (UV) light, oxygen, and renewable raw materials to provide more sustainable manufacturing and broader flexibility into a variety of markets.
While considered a positive leap forward, even this LEGO-like approach to “wrangling molecules” has side effects that do not always move chemical reactions or material properties into the desired manifestations. Unintended byproducts and surprise reactions between the wrong molecules often impede the formation of the desired product.
Add to these issues the complexity of inefficient multistep reactions, all of which must be forced into a precise order, and watch many promising endeavors die on the vine. Chemical complexity demands large amounts of human and monetary resources usually not allocated for long-term research.
The ideal reaction would be one that is flexible, quick, reacts independently of added incentives (heat, UV, etc.), does not interfere with other constituent molecules, and still provides the desired effect. The answer to this wish list could well be click chemistry.
What is Click Chemistry?
Around 2001, shortly after winning his first Nobel Prize in chemistry, Karl Barry Sharpless coined the concept of click chemistry—a new minimalistic approach to molecular formation. He argued that it was somewhat fruitless to try to imitate the creation of natural molecules (the basis of chemical reactions for all time) because their construction was too inefficient and difficult to master.
Instead, Sharpless defined a functional form of chemistry in which building blocks snap together quickly and efficiently. The key to success was to bypass the need to artificially force resisting carbon atoms from different molecules to form bonds with each other. The big problem, aside from difficulty, was that artificially activating these reactions led to unwanted bonds and side reactions that devoured much of the material. Consequently, very little useful product was often generated from these complex, expensive reactions.
Around the same time, Morten Meldal independently discovered what is now known as the crown jewel of click chemistry: the copper-catalyzed azide-alkyne cycloaddition.[1] This reaction is now used globally to link molecules together in a variety of product disciplines. Figure 1 presents a visual schematic for one of the most prolific and clean reactions in click chemistry.
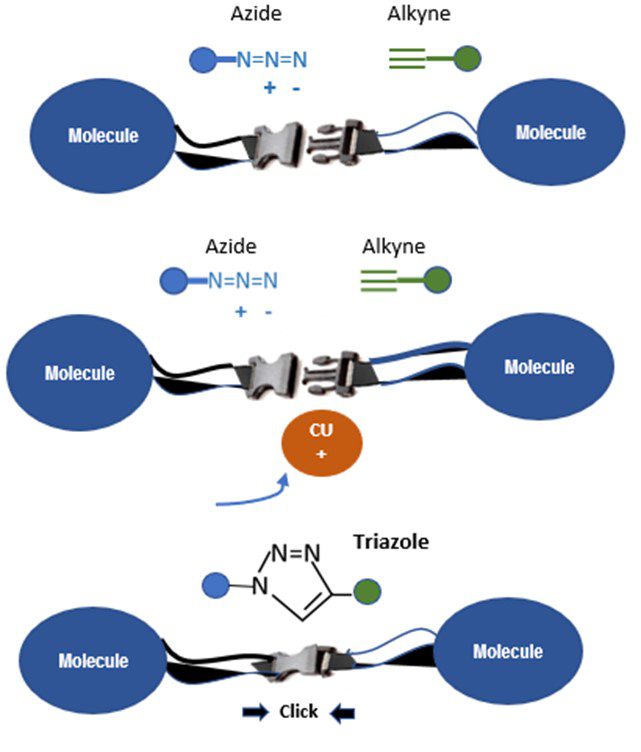
Ironically, this groundbreaking reaction was a happy accident. While trying to run a routine reaction with an alkyne and an acyl halide using some copper and palladium for catalysis, it was discovered that the alkyne reacted to the wrong end of the acyl halide molecule. Further, copper ions controlled the reaction so that only one substance was formed. Even the acyl halide, which should have bonded to the alkyne, remained untouched. What actually happened was that the azide reacted with the alkyne to create a ring-shaped triazole, as shown in Figure 1.
This was a huge deal because triazoles are very stable and found in dyes, agricultural chemicals, and pharmaceuticals. Trying to create triazoles “from scratch” led to unwanted byproducts and significant material waste. This new click reaction was further found to bond together numerous different molecules in a diverse assortment of product disciplines.
Click Chemistry Advancements
To date, the most numerous advancements in click chemistry have occurred within the pharmaceutical and medical industries. These disciplines are well funded and are always looking for new, more efficient, and less invasive ways to treat a range of illnesses—including cancer—while endeavoring to generate fewer side effects.
Most recently, Carolyn Bertozzi developed bioorthogonal reactions in living organisms by taking advantage of strain-promoted alkyneazide cycloadditions to track glycans. This was a further breakthrough in the relatively new science of click chemistry. The copper-catalyzed reaction mentioned earlier as the crown jewel of click chemistry is toxic in living organisms—so it cannot be used for treatment reactions.
First, Bertozzi modified the Staudinger reaction by connecting a fluorescent molecule to the azide introduced to the cell glycans without adversely affecting cells. Next, she took advantage of the 1961 fact that azides and alkynes can react explosively without the help of copper if forced into ring-shaped structures. This strain-promoted alkyne-azide clycloaddition is now used to map cell functionality without disturbing normal cell chemistry.
Bertozzi’s work is leading researchers to explore targeted cancer treatments that shut down the ability of the tumor to protect it from a human immune system. This is done by joining a glycan-specific antibody to enzymes that break down those protective glycans on a tumor surface. Clinical trials are currently underway and, if successful, represent a huge stride in the quest to cure cancer.
The main objective in click chemistry is function. Success means that a variety of material disciplines can utilize molecular chemical interactions and manipulate optimization of useful properties. Twenty-three years after the breakthrough by Sharpless and colleagues, click chemistry is gaining a strong foothold in the development and, more importantly, adoption within those materials. And everything is not necessarily new—but rather a revisiting of old chemicals and processes that have been found to be useful in metal-free click reactions that are now compatible with biological systems.
The second objective for click chemistry is to make reactions “easy to do” for all the disciplines where researchers are not trained as chemists. This means that “clicking” is beneficial for many applications beyond physics, chemistry, biology, material, and polymer science.[2]
As click chemistry evolves, more and more researchers—and even highly sophisticated chemists—are content to leave the carbon-carbon linkage formation to Mother Nature. They are finding instead that, similar to the glycan work performed by Bertozzi, it is more useful to focus on much quicker and easier reactions than those that nature uses infrequently. The structures that we can form through click chemistry by opening aziridines and epoxides are novel concepts—and the assembly sequence could be more than one such reaction. This is essentially what Bertozzi did with her strain-promoted alkyneazide clycloadditions.
Another clever property of click chemistry reactions is the ability to disconnect the reaction on command. This is known as “clip chemistry” because breaking bonds can be just as important as forming them.[3]
Edging closer to the coatings front, significant work has been done with reaction sequences for epoxide ring-openings with hydrazine and subsequent condensation with ß-dicarbonyls or other bis-electrophiles to generate an enormous range of heterocyclic structures for potential reactions. Library synthesis of these molecules is highly efficient, providing a wide base of potentially compatible reactants. A huge diversity within the large, available pool of epoxides gives researchers wide latitude in selecting reactions for material modification, improvement, and customization.[4]
Click Chemistry in Coatings
Coatings offer an attractive area of interest for click chemistry, particularly with the myriad almost-contradictory objectives of cost reduction, greener technologies, and greater durability. In 2008, click chemistry began synthesizing linear polyurethanes (PU) with alkyne groups located on the backbone by reacting two different alkyne-functionalized diols (PDM and DPPD) with a diisocyanate compound.[5]
These PUs generated multiple “clickable” functions that resulted in high thermal stability and improved char yield that was proportional to the alkyne content without interfering with the basic PU chemistry. If making use of readily available azide compounds continues to be successful, universal functionalized PU will generate new classes of PU coatings with easily adaptable physical properties.[5]
Next, the Huisgen 1,3-dipolar cycloaddition was used by reacting these alkyne functionalized materials with several azide compounds in the presence of a copper catalyst. The successful click reaction with BzN3 allowed amine and difluorinated compounds to attach to PU, leading to good yields and new functionalities in the side-chains attached to the backbone.
Click chemistry offers many advantages for the modification of biomaterials, surfaces, particles, and organic compounds. Those advantages include: broad applications, modularity, mild reaction conditions, reliability of both small- and large-scale reactions, high yields, little to no need for purification, inoffensive byproduct generation that is highly desirable in green technologies, and compatibility with living systems for targeted cell treatments.
Out of the nine different types of click reactions, three are most heavily used in biomaterials from simple biolabeling and detection to advanced Clustered Regularly Interspaced Short Palindromic Repeats (CRISPR) applications.
The top nine current applications taking advantage of click chemistry include: biomolecule labeling and detection; solid- and solution-phase biomolecule modification/ligation; construction of analog compound libraries; in situ click chemistry for drug lead discovery; drug delivery; and material optimization in the areas of polymer modification, probes for viral studies, CRISPR sgRNA synthesis, and target gene labelling and novel applications like “click to release.”[6]
In 2016, click chemistry was introduced into waterborne systems as a crosslinking strategy using reactions between copper sulfate and sodium L-ascorbate (Figure 2).[7] These coatings exhibited films with significantly improved tensile strength, hardness, adhesion strength, water resistance, and solvent resistance compared to traditional water-based polyurethane coatings.
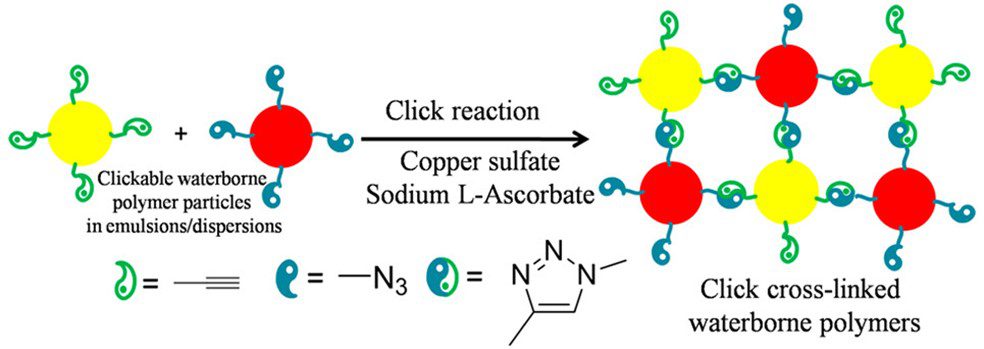
These click chemistries as alternatives to other available crosslinking strategies are not only broadly applicable to PU chemistries, but also to polyester dispersions and polyacrylate emulsions—without generating small molecule byproducts. Selfcrosslinking systems based on N-methylolacrylamide, pendent acetoacetate groups and reversible keto-hydrazide reactions cannot be applied universally to all three polymer systems.[7]
It also appears that those improved film properties can be achieved through click crosslinking without the need for traditional hardeners. The ability to eliminate components and additives in paint systems without damaging physical properties has the potential to reduce costs and counteract supply chain issues when manufacturing industrial coating applications.
Functional Chemistry
Sharpless, Meldal, and Bertozzi shared the 2022 Nobel Prize in chemistry for laying the foundations of click chemistry and bringing chemistry into an era of true functionalism. The discoveries were elegant, clever, and novel and have given rise to applications that are useful—the latter adjective being the most important outcome for two-time Nobel Prize winner Karl Barry Sharpless.
For more information, contact the author at cgosselin@chemquest.com.
References
1. Fernholm, Ann, “Their Functional Chemistry Works Wonders,” The Royal Swedish Academy of Sciences, The Nobel Prize in Chemistry 2022.
2. Castelvecci, D. and Ledford, H., “Chemists Who invented Revolutionary ‘Click’ Reactions Win Nobel,” Nature, 610, 242-243 (2022).
3. Devaraj, Neal K. and Finn, M.G., “Introduction: Click Chemistry,” Chem. Rev., 2021, 121, 6697–6698.
4. Sharpless, K.B., et.al., “Click Chemistry: Diverse Chemical Function From a Few Good Reactions,” Agnew. Chem. Int. Ed., 2001, 40, 2004-2021.
5. Fournier, D., and Du Prez, P., “‘Click’ Chemistry as a Promising Tool for Side-Chain Functionalization of Polyurethanes,” Department of Organic Chemistry, Polymer Research Group, Ghent University, May 1, 2008.
6. “The Top 9 Click Chemistry Life Science Applications You Need to Know,” J&K Scientific LLC website, https://www.jk-sci.com/blogs/resourcecenter/ the-top-9-click-chemistry-lifescience- applications-you-need-toknow (accessed January 20, 2023).
7. Hu, Jianging, et.al., “Click Cross-Linking- Improved Waterborne Polymers for Environment-Friendly Coatings and Adhesives,” ACS Applied Material Interfaces, 2016, 8, 27, 17499–17510.
See the article in CoatingsTech.