Table of Contents
Anyone who has had the pleasure of scraping ice off a car windshield, looking nervously at beautiful but deadly ice spears hanging from winter rooftops, or waiting on a slow and interminably long aircraft deicing line at an airport has dreamed of having some sort of coating that would eliminate inconvenient ice and snow accumulation. The desire to triumph over ice and snow accumulation has, in fact, been the focus of significant technical work that has been going on since the 1940s in an attempt to make all kinds of surfaces ice and snowphobic.
What are Icephobic Coatings?
Icephobicity is defined as the ability of a solid surface to repel ice or prevent ice-film formation due to topographical structures at the ice-surface interface. Ice-repelling characteristics are attributed to the surface structure (sometimes called surface roughness) and/or low surface energy leading to poor ice adhesion, allowing for easy removal.
Icephobic coatings are formulated to ensure that ice doesn’t accumulate on or strongly attach to a surface. In fact, fully functional icephobic coatings operate on several different levels. They can have the ability to repel water droplets, delay ice nucleation, and/or reduce ice adhesion.
Icephobic performance is evaluated using two comparative test methods. The ice Centrifuge Adhesion Test (CAT) measures the adhesive failure between the ice and the surface–in other words, the force required to separate the ice buildup from the coating is measured. In this test, a bare aluminum reference and a coated aluminum sample are simultaneously iced by supercooled precipitation. The ice-adhesion shear stress is calculated from the ice-detachment rotation speed. The results are reported as the Adhesion Reduction Factor (ARF), which is the ratio of ice-adhesion stress on the bare aluminum and the ice adhesion stress on the coated sample.
The second test method is the Static Ice Accumulation Test (SAT), which measures the amount of ice that accumulates on uncoated reference and coated substrates that are placed at angles of 45° and 80°. The results, reported as the Accumulation Reduction Factor (ACCRF), are the ratio of the ice mass on the bare reference sample over that of the coated sample.
Fully functional icephobic coatings operate on several different levels. They can have the ability to repel water droplets, delay ice nucleation, and/or reduce ice adhesion. Over time, a wide range of ARF values (from 0.5 to 1000) have been reported that correlate to interfacial stresses of 800 kPa and 0.5 kPa, respectively.[1]
Which Properties are Important to Icephobicity?
Several properties influence icephobicity. Hydrophobicity in particular prevents water droplets from attaching to and penetrating through the surface. Hydrophobicity is a function of water-contact angle. As shown in Figure 1, three levels of hydrophobicity are defined for contact angles >90°.
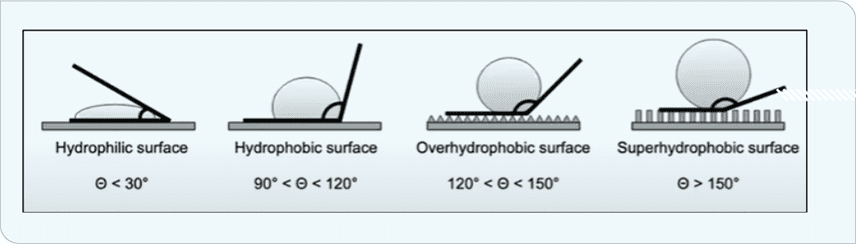
Superhydrophic surfaces (Figure 2) have strong water-repulsion properties that also decrease ice-nucleation temperatures or increase time to ice nucleation, leading to lower ice-adhesion strength.
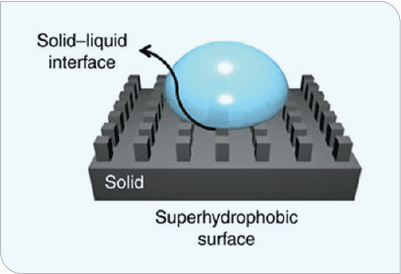
The reduction of ice-adhesion strength, known as interfacial cavitation, is the critical factor in determining the force in removing an ice crystal from the surface. This force is not trivial, as ice adhesion can be as high as 1,600 kPa on an untreated surface. Conversely, icephobic surfaces exhibit ice-adhesion forces of 100 kPa or less. Figure 3 illustrates the forces necessary for ice detachment. Detachment mode, shear-mode deformability, and tensile-mode hydrophobicity all impact the adhesion strength. Oil infusion further reduces ice adhesion due to interfacial slippage.
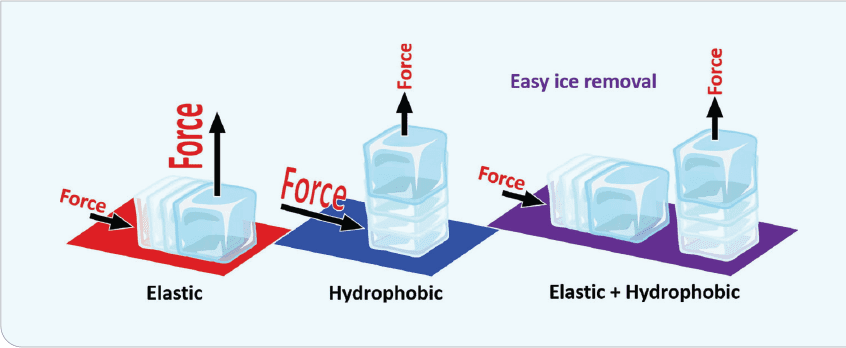
The concept behind interfacial cavitation is that a soft surface (polymeric elastomer, for example) attaches to a hard surface (ice). The elastomer deforms while the ice remains a hard, inflexible mass, causing a stress concentration at the interface and thus making the ice break free. This was developed accidentally when it was discovered that different adhesion strengths can be tailored for the same polymer. Adhesion strengths as low as 0.1 kPa can be achieved with some of these coatings–although at that level, they may be too fragile.
Lubricants, greases, and gels have very low ice-adhesion strength on the order of 0.4 Kpa—but are sacrificial coatings and are not durable enough to last more than a few cycles without being replenished. An example of this type of application, where it is expected that coatings are reapplied often, is in consumer recreational for sleds, toboggans, and saucers.
Elastomers, on the other hand, can exhibit both solid and liquid-like properties. To reduce the force required to remove ice from the surface, oil-impregnated polymers are coated onto the surface, providing durability and mechanical and chemical stability with very low ice affinity. These Slippery Liquid Infused Porous Surfaces (SLIPS) have low-contact-angle hysteresis and low sliding angles, causing excellent liquid repellence.
First, these elastomers are made softer by decreasing the crosslink density. For example, polydimethylsiloxane (PDMS) that is heavily crosslinked exhibits ice-adhesion strength of over 250 kPa. By reducing the crosslink density, ice adhesion strength as low as 33 kPa can be achieved. Interfacial cavitation requires that tiny air pockets form between the hard ice and the soft rubber. The softer the rubber, the easier air pockets can be created by flexible movement of the polymer.
Second, adding oil to the various elastomers allows lightly crosslinked chains to move more freely. Adding oil to even highly crosslinked PDMS reduces the adhesion strength from 264 kPa to 58 kPa. Combining a lower crosslink density with oil further reduces the adhesion strength of ice to a low of 6 kPa–the lowest level ever achieved using a practical coating. Even with the oil impregnation, the surface of the coating remains dry to the touch.
Icephobicity—and to some extent, SLIPS—is maintained over a wide range of temperatures primarily due to the presence of the liquid lubricant infused into the coating. These types of coatings can be applied using standard industrial processes. This could be a viable solution for many applications across industrial, military, and consumer landscapes for parts that are not easily accessible during routine maintenance. The <15 kPa adhesion strength exhibited by these coatings is well below the 100 kPa defining limit of icephobic materials.[5]
This work has generated a library of reduced crosslink density oil-impregnated polymers. Some are too soft to use in today’s applications, despite very low kPa strength levels. However, there are now a series of polymeric choices that can be added to coatings exhibiting an order of magnitude lower adhesion strength than commercially available icephobic coatings. The added advantage is that many of these materials have durability good enough to use on wind turbines and aircraft. Examination of the underlying polymer physics and fracture mechanics of molecular chain movement under ice conditions will generate even more reliable coatings specific to many service environments.[6]
Physically texturing grooved surfaces, which traps air and prevents water from freezing when contacting the surface, was effective in the laboratory (and on penguins). However, in real-world applications, this proved to be ineffective because the air channels became clogged with frost.[7] Another icephobic surface works by using latent heat released by droplets as they freeze to propel them away from the surface. Unfortunately, this only works well below atmospheric pressure–so not a very practical solution.[8]
A supercooler is a heat exchanger that supercools water, thereby avoiding ice nucleation on the heat-exchanger surface. Ice storage is used for multiple applications in residential, industrial, and commercial refrigeration. In order to improve the performance, four different chemical families of coatings were tested:
- Hybrid organic/inorganic siloxane sol gel modified with additives to form a hydrophobic and oliophobic surface
- An icocyanate-free silicone-epoxy coating matrix
- Methyl polysiloxane resins with silicon oil
- Fluoroethylene polymers with hydrophobic additives
All of the coatings were able to improve the performance of the supercooler by 7-44% over the uncoated equipment. The best improvement was shown by the hybrid organic/inorganic siloxane sol gel, with 30-40% greater supercooling performance. With heightened awareness of energy consumption, this is a viable option for improving supercooling performance without additional energy requirements.[9]
Developing Technologies for Icephobic Coatings
Greases, lubricants, sol gels, SLIPS coatings, and macro- and micro-surface texturing have been under development to solve ice accretion for more than 75 years. Yet none of these technologies have proven entirely satisfactory, despite the fact that 22 companies have focused entirely on developing commercially viable products. The myriad industries that benefit from icephobic coatings technology span aviation, medical, industrial, wind turbine, marine, arctic infrastructure, HVAC, residential, and recreational enterprises. And it seems that, as of yet, no single solution is appropriate for all applications.
A totally different approach, albeit a non-coatings one, was taken by the Nanotherm Research Department at the University of Houston Cullen College of Nanotechnology. The low interfacial energy of magnetic liquid-liquid interfaces with exceptional icephobicity, unprecedented low ice-adhesion strength, negligible friction allowing for outstanding SLIP properties, self-healing characteristics, and high shear-flow stability generated another avenue for generating icephobicity (Figure 4).[3] In this case, a magnet was placed beneath a copper surface that was coated with ferrofluid. Water was applied to the surface and allowed to freeze. These magnetic slippery surfaces (MAGSS) provide a defect-free ice nucleation surface, reducing ice formation to close to the homogeneous nucleation limit.
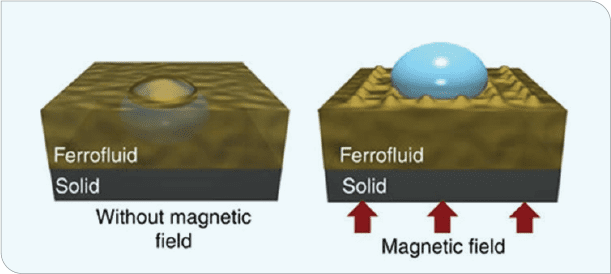
All of the coatings were able to improve the performance of the supercooler by 7-44% over the uncoated equipment.
Ice-formation temperature is reduced to -34°C (-29°F), allowing for two to three orders of magnitude longer of ice-formation delay. Further, this surface exhibited adhesion strengths of approximately .002 kPa, five orders of magnitude lower than conventional coatings. The volumetric force exerted by the magnetic field does not allow formation of a solid-water interface (Figure 5). Ice-adhesion strength is so low that tilting the sample a mere 2.5° allows ice to easily slide off the surface.
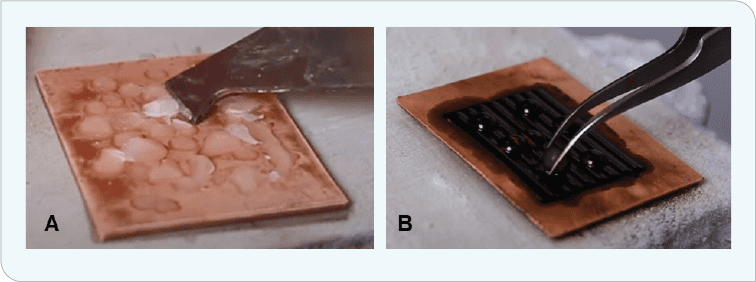
Figure 5 (B). Placing a magnet beneath the copper plate and coating the top with ferrofluid delayed water freezing and reduced the ice/surface interfacial strength to the point that ice was easily removed from the surface (or slid off if the panel was tilted even 2.5°).[10]
This development, which requires no nanotexturing of the surface, is a unique paradigm that could provide icephobic capability for applications often positioned in extreme environments, such as aviation, marine industries, power lines, and wind turbines.
Understanding Snowphobicity
One additional complexity when considering icephobicity is that of snowphobicity. They are not quite the same, as shown during field testing in the Italian Alps, although the goal of both is to reduce or eliminate accumulation of frozen water onto a surface.[11] In snowphobicity, the first order effect is to reduce the accumulation of snow. If the surface can remain free of snow for a longer time, less egregious accumulation is likely to occur.
Some coatings allow for frequent “shedding phases,” while others allow for snow detachment at a critical weight accumulation. The physical structure of the surface will have an effect on which mode is preferred.
An anti-snow effect can occur even if the coating does not have anti-ice properties, making icephobicity a sufficient but not necessary condition. Rather, the mobility of water on the coating surface at low temperatures may be more important. Coatings with low-temperature SLIPS surfaces allow a snowflake with 15-20% water content to more easily slide off and detach from the surface–regardless of snow accumulation.
Coatings with different dynamic ratios (sliding angle/roll-off angle ratios) at low temperatures exhibit different behavior under wet-snow or dry-snow conditions. This may be due to the low sliding angle, which is maintained at temperatures as low as 0°C, allowing better sliding-off of wet snow at the beginning of the weather event. At lesser dynamic ratios, wet snow is likely to become trapped in the nano-rough surface–leading to quicker accumulation.
Continued Development
It appears that after 80 years of development, icephobic coatings have only scratched the surface. However, the search continues, with many promising alternatives on the horizon.
For more information, contact the author at cgosselin@chemquest.com.
References
- Laforte, C.; Blackburn, C.; Perron, J. A Review of Icephobic Coating Performances over the Last Decade. SAE Technical Paper 2015-01-2149, 2015.
- American Concrete Institute. “Superhydrophobic, Ice-Phobic, and Photocatalytic Coatings for Concrete.” YouTube, May 26, 2022. https://www.youtube.com/watch?v=eWvOWHxdtBU (accessed November 9, 2023).
- Irajizad, Peyman, et al. (2016). Magnetic Slippery Extreme Icephobic Surfaces. Nature Communications, 7, Article number 13395.
- Ibáňez-Ibáňez, F., et al. (2022). Ice Adhesion of PDMS Surfaces with Balanced eEastic and Water-repellant Properties. Journal of Colloid and Interface Science. Volume 608, Part 1, 729-799.
- Wogan, T. Best Icephobic Coating Ever Created Gives Ice the Slip. Chemistry World, Royal Society of Chemistry, March 20, 2016.
- Kota, A.K., Li, Y., Mabry, J.M. and Tuteja, A. (2012), Hierarchically Structured Superoleophobic Surfaces with Ultralow Contact Angle Hysteresis. Adv. Mater., 24: 5838-5843. https://doi.org/10.1002/adma.201202554
- Stoye, E. Penguin Feathers Ice Resistant Design Revealed. Chemistry World, Royal Society of Chemistry, February 23, 2016.
- Extance, A. Trampolining Droplets Raise Hopes for Ice-shedding Surfaces. Chemistry World, Royal Society of Chemistry, November 3, 2016.
- Carbonell, D., et al. (2022) Development of Supercoolers for Ice Slurry Generators Using Icephobic Coatings. International Journal of Refrigeration. 144,90-98.
- University of Houston Engineering. “Magnetic Slippery Extreme Icephobic Surfaces.” YouTube, November 9, 2016. https://www.youtube.com/watch?v=eWvOWHxdtBU (accessed November 9, 2023).
- Balordi, M., et al. (2023) The Anti-snow Behavior of Icephobic Coatings: Laboratory and in-field Testing. Coatings, 13, 616.
Read in CoatingsTech.